Milk intake, body anatomy and composition in calves
Dominique Pomies 2
Volker Röttgen 1
Björn Kuhla 1
Stefan Nüske 3
Alex Bach 4
Armin M. Scholz 3
1 Leibniz Institute for Farm Animal Biology (FBN), Dummerstorf, Germany
2 Université Clermont Auvergne, INRAE, Saint-Genès-Champanelle, France
3 Ludwig Maximilian University of Munich, Oberschleissheim, Germany
4 Institució de Recerca i Estudis Avançats (ICREA), Barcelona, Spain
Introduction
Calves are an important investment on a cattle farm as their loss can cause substantial financial damage [1], [2]. Young calves are susceptible to a range of different diseases caused by pathogens, which can lead to morbidity and mortality [3]. Additionally, calf management, including cow-calf separation, colostrum feeding, housing, dehorning and vaccination, can directly impact the health of calves [4]. This chapter is therefore dedicated to different methods for assessing specific traits in calves.
In the first few days following birth, calves do not have a fully functioning rumen, i.e. they are pseudo-monogastrics, hence nutrients are fed to them mainly in liquid forms such as whole milk (WM) or milk replacers (MR). The current focus in calf rearing globally is optimal growth performance; therefore, WM or MR with a high nutritional value is offered to calves [5]. Higher average daily gain (ADG) in the first weeks of a calf's life results in higher body weight at 24 months of age [6], promoting gastrointestinal development and reducing the age at first calving [7], [8]. Whole milk contains several non-nutritional bioactive compounds, such as hormones and growth factors in comparison to commercially manufactured MR [9]. However, feeding MR greatly reduces the potential transmission of diseases such as Johne's disease, which disturbs the health and productivity of the animal [10]. Different restricted and intensive milk feeding regimens have been applied to pre-weaned calves throughout the past years [11], [12]. Restricted milk feeding tends to promote concentrate intake pre-weaning [13]. Nevertheless, this feeding approach is associated with lower ADG in pre-weaned calves, higher calf mortality, impaired rumen development, and abnormal behaviours [14], [16]. Intensive milk feeding planes have resulted in changes at the cellular level in the mammary gland, which in turn may have depicted a long lasting effect on the future milk production capability of the animal [17]. Calves receiving higher levels of WM or MR during the pre-weaning period are less prone to detrimental diseases [18], [19]. Manual feeding is considered the conventional method of milk feeding, where animals are generally kept in individual pens (up to 8 weeks of age) and fed via open buckets or bottles [20]. Manual feeding is often considered a laborious task. However, individual restraining of calves could disrupt their welfare and behaviour and subject them to possible hunger and frustration [21], [22]. Automated milk feeders provide an alternative to the manual approach by exploiting computer-based systems that enable group housing of calves, yet at the same time monitor individual milk intake. It is important to record individual milk intake, as deviations between allowance and true milk intake may provide insight into animal health. Despite the positives associated with automated feeders in group stalls, appropriate management programmes must be implemented to prevent calves being exposed to disease due to intensified group housing [23].
Developing techniques to accurately measure body composition in cattle for various applications, such as breeding programs or studies on growth performance, has gained more interest among researchers in recent years [24]. Previously, the most common approach to body composition analysis was to slaughter the animal and assess the whole tissue [25]. Despite providing accurate results, this method requires the animal to be sacrificed. Additionally, this method does not necessarily present an unambiguous outcome if the aim of the study is to monitor continuous growth in the animal [26]. Therefore, it is essential to develop non-invasive methods capable of producing precise data on live animals, thus allowing body composition of the same animal to be assessed at different stages of its life. Scholz [24] stated that the key characteristic of such non-invasive methods for body or carcass composition measurement is to exploit electromagnetic or mechanical energies, which are able to pass completely or partially through body tissues e.g., muscle, adipose tissue and bone. An important advantage of such novel imaging techniques is the ability to introduce new traits which can be directly incorporated in future phenotyping programs. However, most of these techniques are relatively new in cattle research, and the data acquired still needs to be compared with a globally accepted reference system (gold standard method). Moreover, techniques such as dual-energy x-ray absorptiometry (DXA), computed tomography (CT) or magnetic resonance imaging (MRI) require the animal to be sedated prior to measurements. Therefore, future studies should focus on assessing whether the sedation process has any impact on the measurements acquired from the animal using these techniques.
Milk intake
Prerequisites
This guideline is intended to provide some steps associated with milk replacer intake in calves. This guideline presumes that automated milk replacer (MR) used to assess individual MR intake. The Animal Trait Ontology (ATOL) and Environment Ontology (EOL) for Livestock numbers associated with the present guideline are:ATOL_0000793, ATOL_0000365, ATOL_0001568, EOL_0001940 and EOL_0001646 (for complete list of ATOL and EOL please visit https://www.atol-ontology.com/en/erter-2/).
A – General points on milk feeding
- Rearing calves requires great dedication from the staff members of the experimental unit with regards to feeding and general health.
- It is highly important to note that calves must receive colostrum during the first 6–12 h after birth, prior to feeding with any type of transition milk or MR. If maternal colostrum is not available, either colostrum from a pool of other cows or frozen colostrum (kept at −20°C) must be fed to calves. Frozen colostrum has to be thawed in warm water (not hot) with a maximum temperature of 50°C and thawing and refreezing of the colostrum must be avoided. Calves should receive between 3–6 l of colostrum during the first 24 h of their life ideally in two or three feedings, though a minimum of 1.5 l must be fed during the first 2 h after birth.
- During the transition from colostrum/transition milk to MR, the volume of MR should be kept in the same range as the milk has been fed at.
- Calf hygiene during the early days after birth should be carefully considered. MR should be freshly prepared and all equipment must be thoroughly cleaned post feeding. MR has to be reconstituted by adding the total amount of powder (based on the manufacturers recommendation) to half the measured volume of water, mixed thoroughly in order to obtain a homogenised suspension and then the remaining balance of warm water (38–41°C) to make up the correct volume.
- MR should be fed to calves at least twice per day (when feeding is done manually) until they are at least 6 weeks of age.
- Unless the intention of the experiment states otherwise, the MR allowance should allow for calves to achieve an average daily gain of 850–1,200 g during the rearing period (8–12 l/d of MR is recommended). MR allowance (% bodyweight) during the rearing period must be adjusted once per week.
- The crude protein and crude fat content of the MR should be in the range of 20–22% (DM) and 18–20% (DM), respectively.
- During cold weather conditions (<10–12°C) the amount of MR feeding must be increased. Generally, the MR amount should be increased by 1–2% for every degree the ambient temperature falls below 10–12°C.
- Feeding MR should be consistent and therefore switching between different MR brands should be avoided.
- Calves less than three weeks of age must be fed an all-milk protein milk replacer. Operatives should consider that non-milk sources can decrease available protein to the calf and result in diarrhoea.
B – Milk replacer auto feeder (MAF)
- Calves' general performance should be monitored through the data acquired on eating behaviour from the MAF system, as well as direct observation.
- The neck transponder or ear tags of the calves should be checked to ensure correct function.
- Fortnightly and bimonthly manual calibration of the mixer scale must be performed for non-auto calibration and auto-calibration MAF, respectively, in order to achieve consistent MR delivery (with few total solid content variations).
- Calibration of the mixer scale must be conducted whenever a new MR batch or brand is introduced to the unit.
- Generally, one nipple on the MAF should be sufficient for a pen with 15–20 calves grouped together. The feeder nipple is required to be set 70–80 cm above floor level.
- The MR powder hopper and storage apparatus of the system should be checked for correct functioning.
- The powder delivery system should be examined at least once a week. The mixing temperature of MR powder within the feeder requires routine monitoring throughout the year (once/month) along with appropriate corrections due to seasonal changes. Additionally, the suction hose along with the nipples of the MAF require frequent examination (at least 3–6 times per week).
- The mixer cleaning cycle should be done 2–3 times per d. The milk powder outlet should be cleaned frequently (4–6 timer per week) to prevent caking. Cleaning of the mixer/heat exchanger should be done at least 4 times per d (automatically by the MAF) in order to reduce bacterial contamination of the system and risk of disease in calves.
- Where a MAF is installed, clean bedding with enough space (minimum 2.8–3.5 m2/calf), along with ventilation systems with a sufficient air exchange rate are necessary to reduce the risk of disease.
- Any calf identified as having abnormal behaviour by the system should be immediately checked for any sign of disease. Diseased animals must be moved to individual pens for further observation and treatment to ensure standard hygiene and welfare conditions are met.
- Data from MAF should be recorded as daily MR intake (l/d or g/d), as well as proportion (%) of the daily allowance. The time at the start and end of each visit, number of unrewarded visits (visits without any MR feeding), along with number of total visits, should be analysed for observing calf milk feeding behaviour.
- Particularly during the first week, calves will require assistance from staff members to adapt to drinking from the MAF.
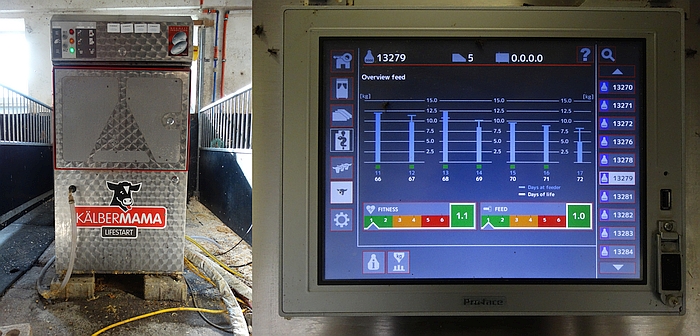
Monitoring rumen development
Prerequisites
This guideline provides steps on observing the rumen development in calves during rearing and weaning via ultrasound. The Animal Trait Ontology for Livestock (ATOL) numbers linked with this guideline are: ATOL_0000582, ATOL_0001061, ATOL_0000583 and ATOL_000585 (for complete list of ATOL, please visit https://www.atol-ontology.com/en/erter-2/).
Ultrasound examination of the rumen
- Ultrasonography examination of rumen development in calves is a useful tool for diagnosing diseases, associated with the digestive tract.
- Ultrasound examination of the rumen can be carried out on calves from 7 d to 7–9 months after birth.
- Examination should be conducted once every 1–3 weeks during trials. Examination of calves should be performed approximately 3–6 h after feeding with milk/milk replacer.
- During examination, animals must be non-sedated and in a standing position.
- Haltering or restraining of the calves must be done carefully without any unnecessary stress towards the animal.
- The examined calf should be clipped on both sides from shoulders to the tubar coxae and from the thoracic and lumbar vertebrae to the ventral midline [27]. Skin must be cleaned with alcohol but use of contact gel for the transducer is optional.
- The ultrasound instrument should contain a sector array or linear or convex transducer with 5–13 MHz, to scan between the ribs of young calves. The penetration depth of the transducer must be in the range of 3–10 cm dependent on the frequency setting of the instrument.
- The rumen should be scanned between the 6th to 12th cranial intercostal space (ICS), as well as the flank at both sides from dorsal to ventral, with the transducer held parallel to the ribs.
- The dorsal and ventral margins can be marked by measuring the distance between each area to the dorsal midline using a tape measure (or alternatively, the margins marked using animal marking crayons). The rumen size should be calculated by subtracting the distance of the dorsal margin from the distance of the ventral margin [28].
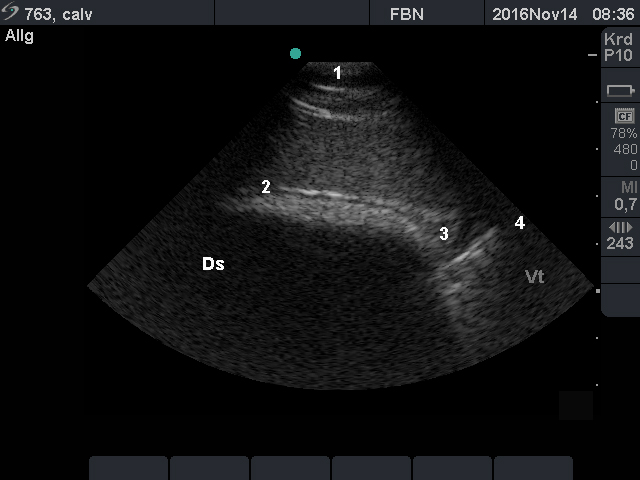
- The location of the longitudinal groove should be determined to assess the size of the rumen sacs.
- For measuring the rumen wall thickness, the operator should examine it near the dorsal margin, the longitudinal groove and the ventral margin, using the transducer with a higher frequency (e.g. 13 MHz).
Mammary parenchyma
Prerequisites
The guideline provides steps for assessing the mammary parenchyma (MP) area in prepubertal calves. This guideline presumes that ultrasound sonography, as a non-invasive tool, will be used to measure the MP region in pre-weaned heifers in vivo. The MP refers to the epithelial tissue and surrounding stromal elements in the mammary gland [29]. The Animal Trait Ontology for Livestock (ATOL) numbers linked with this guideline are: ATOL_0000132 and ATOL_0000537 (for complete list of ATOL, please visit https://www.atol-ontology.com/en/erter-2/).
Steps in MP measurement
- Growth of MP can be monitored by a weekly (once per week) ultrasound examination of the animals during the rearing period (2–3 months after birth). However, possible data variations, particularly during the early weeks after birth, must be taken into consideration.
- A real time B-mode ultrasonograph with a 6.5–7.5 MHz convex probe is required for this measurement.
- The udder is not required to be clipped of excess hair for examination.
- Animals should be laid dorsally with a slight tendency to the lateral left side. Manual restraint is sufficient for this examination.
- The MP area of all 4 glands should be assessed. The examination should follow a similar pattern during the experiment, e.g., starting from right front and moving to right rear, left front and left rear.
- The examination should be performed by an operator/veterinarian with expertise in ultrasound sonography.
- The examination should be performed by the same operator during the trial. If a change in examiner is necessary, the results of the two operators should be compared for any variation at the end of trial.
- Ultrasound gel should be applied to each gland to enhance contact with the probe.
- When the calves are laid down, the probe should be placed directly on top of the teat. As applying pressure will fold the teat, the probe therefore needs to be positioned so that the base of the teat appears consistently on the right side of the ultrasound's screen. Alternatively, the transducer should be applied to the base of each teat at a 45° angle, in a caudal-to-cranial placement as described by [30].
- The operator should take a minimum of 2 images from each gland to allow a choice of the image with the best MP definition for further analysis.
- Staff members should be aware that the MP region is hypoechoic (black) on ultrasound when compared with the mammary fat pad, which is hyperechoic (white). Quantification of the regions from the images should be done with appropriate software, such as ImageJ (National Institutes of Health, see: https://imagej.nih.gov/ij/index.html).
Body composition evaluation using dual-energy X-ray absorptiometry (DXA)
Prerequisites
There are some assumptions that should be carefully considered prior to following this guideline. This body composition (BC) measurement guideline is intended for use in experimental units where a dual-energy X-ray absorptiometry device is available for calves (calves up to an in vivo body weight of 150 kg). A separate BC measurement guideline by using magnetic resonance imaging will be made available, though no routine MRI body composition measurements have been performed on calves so far. This guideline has been written based upon the assumption that this BC measuring method is utilised only for calves, as the equipment is not suitable for heifers or cows. The Animal Trail Ontology for Livestock (ATOL) numbers linked with this guideline are: ATOL_0000090, ATOL_0000012, ATOL_0000351, ATOL_0000505 and ATOL_0000350 (for complete list of ATOL, please visit https://www.atol-ontology.com/en/erter-2/).
A – Definition of body composition for DXA measurements
- Unless defined otherwise, body composition with DXA measurements follows a three-compartment body composition model, and is defined as mass (in g) or percentage of soft tissue and bone mineral (tissue). Soft tissue is divided into fat tissue and lean tissue. Lean tissue covers all non-fat tissues and all non-bone mineral ‘tissues’. The sum of bone mineral, soft fat tissue, and soft lean tissue provides the total tissue mass or the live body weight for in vivo measurements.
- An estimate for the in vivo whole body muscle (meat) mass may be derived by the following general formula:
Muscle mass (whole body) = total tissue mass × cut out proportion − fat mass − bone mineral mass.
This requires information about the potential cut out proportion of the calf under investigation – mainly based on body weight, breed, sex, and feeding – varying between 0.55 and 0.65 in calves. - The protein mass can be estimated according to the formula:
Protein mass = lean mass × protein content (protein content = 0.2).
B – Basics of a DXA analysis
- DXA measurements are based on the mass attenuation of X-ray photons which differs dependent on the type of tissue(s) and the energy level of the X-ray photons at the source of X-ray emission. Usually, two energy levels (high and low) of the X-ray photons lead to tissue dependent X-ray attenuation coefficients [31].
- Very high X-ray attenuation coefficients (R values) define bone (mineral), and lower ones define soft tissue. A first segmentation step defines bone (mineral containing) and soft tissue. In all non-bone containing pixels (picture elements), a second segmentation step defines fat and lean tissue, with fat tissue having the lowest soft tissue X-ray attenuation coefficient.
- Usually, the relationship between soft tissue X-ray attenuation coefficients and the fat tissue proportion (%) of the tissue (whole body) is linear, but not for very lean animals like young calves.
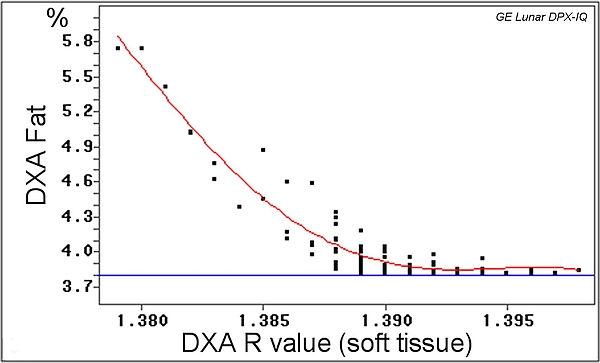
- In addition to whole body analysis, DXA also allows for a regional analysis depending on the region of interest defined.
- Not all devices and manufacturers provide the X-ray attenuation coefficient.
C – Measurement
- Prior to each measurement day, the device needs to run a quality control, based on a device specific phantom.
- Calves must be sedated, for example, by using intramuscular application of Xylacine with 0.2 mg/kg body weight. Dependent on the application, Xylacine is used for slight sedation up to long sedation with muscle relaxation. A dosage of 0.2 mg/kg bodyweight – 1 ml of Xylazine 2%/100kg – leads to stable sedation and loss of energy. Thus, short examinations up to 20 min can take place and restraining the calf on the X-ray table is easy. The dose can be raised to 0.3 mg/kg bodyweight – 1.5 ml/100kg – to reach longer and deeper sedation.
- Calves should be positioned on the scan table in a prone position for a whole-body scan as shown in Figure 2.
- Hind legs should be tight together leaving a small gap between both legs.
D – Validating and analysing the records
- Prior to each scan day, the DXA system requires a 'quality control' scan with a device-specific 'phantom' which contains materials with defined X-ray attenuation coefficients, as standard for high, medium, or low fat percentages and bone mineral densities. Only if the 'quality control' scan is passed, can a measurement scan be performed.
- DXA systems provide several scan modes depending on the 'diagnostic' purpose and on the scan subject itself.
- Whole body scans for the determination of body composition are available as adult or paediatric mode, with different settings depending on subject height like (standard or thick or large, medium, small). Additionally, a small animal mode may be available, but it is unlikely to be suitable for calves.
- DXA body composition and bone mineral results will be available immediately after the scan is finished. Whole body or regional results will be provided (Figures 3 and 4). A regional analysis requires human action by exact definition of the standard 'human' anatomical regions or by a custom definition of body 'regions of interest'.
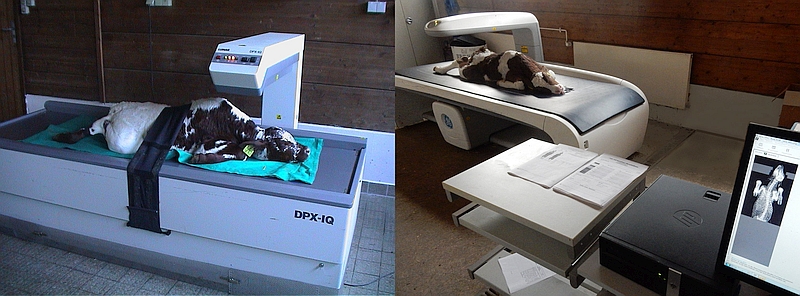
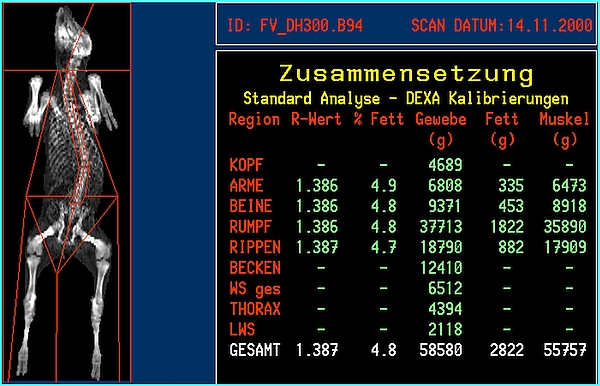
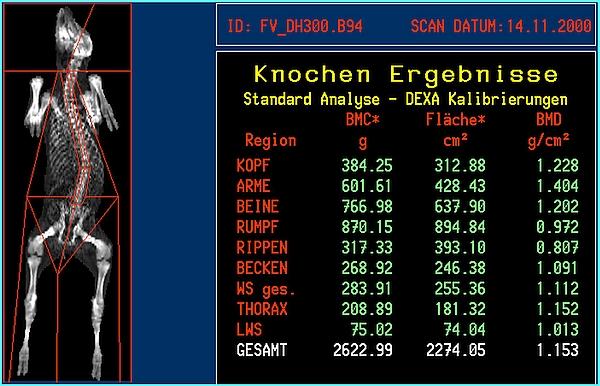
Body composition measurements using magnetic resonance imaging (MRI)
Prerequisites
This body composition (BC) measurement guideline is intended for use in experimental units where a MRI device is available for animals (calves up to a body weight of about 150 kg in vivo). A separate BC measurement guideline for DXA is also available, as currently no 'routine' MRI BC measurements have been performed on calves. The present guideline has been written based upon the assumption that the method is utilised only for calves, as the equipment in not suitable for heifers or cows. The Animal Trail Ontology for Livestock (ATOL) numbers linked with this guideline are: ATOL_0000090, ATOL_0000012, ATOL_0000351, ATOL_0000122 and ATOL_0000520 (for complete list of ATOL, please visit https://www.atol-ontology.com/en/erter-2/).
A – Definition of body composition for MRI measurements
- Unless defined otherwise, BC measurements using MRI are usually based on signal intensity differences of body tissues, such as fat, muscle or bone, dependent on the electro-magnetic proton-proton or proton-non-proton interaction, and differences of the proton densities, defined as volume (in mm³) or area (mm²) of the tissue or organ of interest [32]. Basic anatomical knowledge is therefore generally required.
- Whole body or regional (organ) analysis often follows the ‘Cavalieri’ approach, as applied in stereology [33], acquiring only, for example, a restricted number of transversal slices and interpolating the tissue volumes for the gaps between two slices (images) with a defined depth. Each pixel (picture element) is a voxel (volume element) with the coordinates x, y, z.
- Knowledge of tissue densities (g/cm³) is required if the tissue mass (in g) is the target of the study.
Tissue mass = Tissue volume × Tissue density (fat ~0.91 g/cm³, muscle ~1.06 g/cm³, and bone ~1.43 g/cm³ [34, 35].
B – Basics of MRI analysis
- MRI measurements are based on the electro-magnetic properties of nuclei with an uneven number of protons and neutrons characterised by an ‘electro-magnetic’ spin of the nuclei under investigation. The defined nuclei (e.g. hydrogen = 1H = proton) precess with a defined frequency (Larmor frequency) in a static magnetic field with a field strength between, for example 0.2 and 7.0 Tesla along the field lines in a parallel or anti-parallel direction. A defined radio (wave) signal matching the 'resonance' frequency of the nucleus or nuclei of interest provides an energy input and leads to tissue dependent signal intensities of the relaxing protons (or other nuclei) after the radio wave signal (sequence) stops delivering energy.
- Tissue-dependent signal intensities vary due to different (net magnetisation) relaxation times (T1 or T2, T2*), affected by spin-spin (T2 = e.g. 1H−1H) or spin-lattice (T1 = e.g. 1H−13C or 1H−31P) interactions.
- A T1-weighted image (usually fat = bright signal and other tissue less bright (grey or dark) signal may be acquired by a short time of (sequence) repetition (e.g. TR <700 ms) and an even shorter time between (spectral signal peak) echoes (e.g. TE <30 ms), which might be modulated by different flip angles (of the nuclei of interest).
- In addition to whole body analysis, MRI is most often used for a regional analysis requiring special (gradient) coils, such as body coil, wrist coil, head coil etc.
- It is important to make sure that the magnetic field is homogenous.
- EKG and/or pulse triggered sequences can improve image quality.
C − Measurement
- Generally, the MRI device does not need a quality control check run prior to the measurement day, unlike DXA.
Occasionally it may be necessary to use a device specific phantom for re-calibration of the system, carried out by an MR service specialist. - Calves must be sedated for MRI, for example, by using intramuscular application of Xylacine with 0.2 mg/kg body weight. Dependent on the application, Xylacine is used for slight sedation or long sedation with muscle relaxation. A dosage of 0.2 mg/kg bodyweight – 1ml of Xylazine 2%/100kg – leads to stable sedation and loss of energy. Thus, short examinations up to 20 min can take place and bedding of the calf on the MRI table is quite easy. This dose can be raised to 0.3 mg/kg bodyweight – 1.5 ml/100kg – to reach longer and deeper sedation.
- For a whole body scan, calves should be positioned on the MRI table in a prone position as shown in Figure 1. Positioning on the side is also possible, though the body symmetry can be disturbed.
- Hind legs should be tight together leaving a small gap between both legs.
D – Validating and analysing the images
- Dependent on the service period, the MRI system requires occasional ‘quality control’ scans with device-specific phantoms containing materials with defined 1H concentrations. Usually, the MRI device is ‘self-calibrating’ (shimming), dependent on the object inside the coil(s).
- MRI systems provide several acquisition sequences depending on the ‘diagnostic’ purpose and the subject itself.
- Whole body or regional imaging for determination of the BC depend on the MRI device and the available (combination of) coils.
- MRI whole body or regional composition will be available only after an intensive manual, semi-automatic or fully automatic image analysis [24], [36], [37] as depicted in Figures 3 and 4.
- Several image analysis software applications are available either commercially, such as 3D doctor (Able Software Corp., Lexington, USA), which is FDA-approved, SliceOmatic (Tomovision, Magog, Canada), Analyze 12/Analyze Pro (AnalyzeDirect, Overland Park, USA), and Synedra View Professional (synedra information technologies GmbH, Innsbruck, Austria) or freely, such as synedra View Personal (synedra information technologies GmbH, Innsbruck, Austria), ImageJ (National Institute of Mental Health, Bethesda, USA), and Mango (University of Texas Health Science Center, San Antonio, USA).
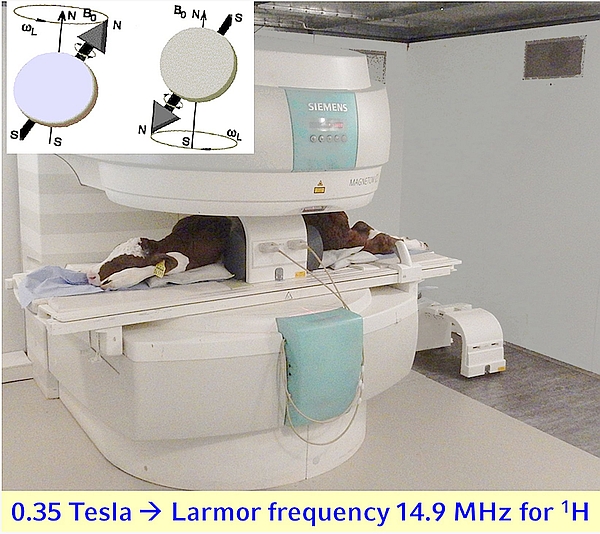
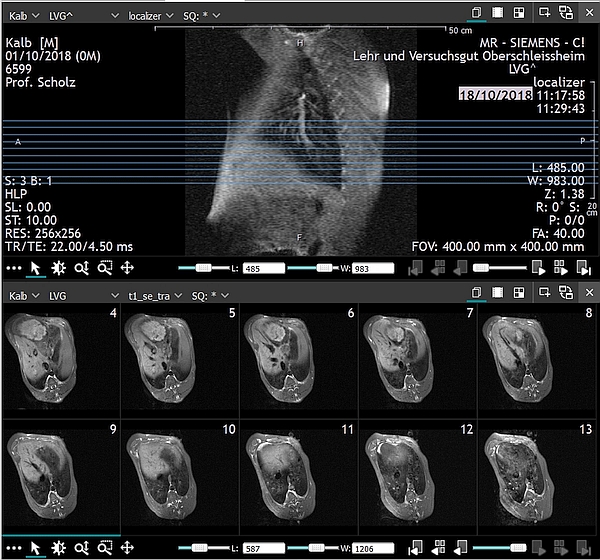
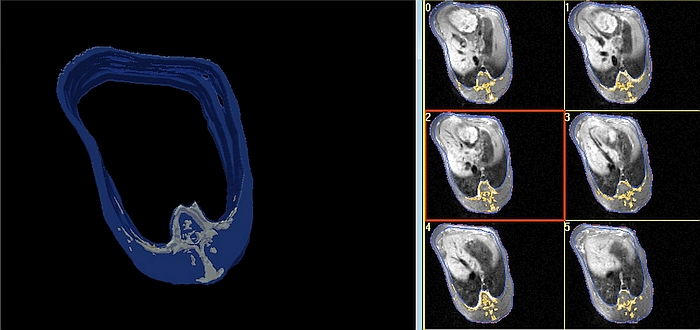
References
[1] Tozer PR, Heinrichs AJ. What affects the costs of raising replacement dairy heifers: a multiple-component analysis. J Dairy Sci. 2001;84:1836-44. DOI: 10.3168/jds.S0022-0302(01)74623-1[2] Wolf CA. Custom Dairy Heifer Grower Industry Characteristics and Contract Terms. J Dairy Sci. 2003;86(9):3016-22. DOI: 10.3168/jds.S0022-0302(03)73900-9
[3] Uetake K. Newborn calf welfare: a review focusing on mortality rates. Anim Sci J. 2013;84(2):101-5. DOI: 10.1111/asj.12019
[4] Pempek JA, Schuenemann GM, Holder E, Habing GG. Dairy calf management—A comparison of practices and producer attitudes among conventional and organic herds. J Dairy Sci. 2017;100(4):8310-21. DOI: 10.3168/jds.2017-12565
[5] Amado L, Berends H, Leal LN, Wilms J, Van Laar H, Gerrits WJJ, et al. Effect of energy source in calf milk replacer on performance, digestibility, and gut permeability in rearing calves. J Dairy Sci. 2019;102(5):3994-4001. DOI: 10.3168/jds.2018-15847
[6] Moallem U, Werner D, Lehrer H, Zachut M, Livshitz L, Yakoby S, et al. Long-term effects of ad libitum whole milk prior to weaning and prepubertal protein supplementation on skeletal growth rate and first-lactation milk production. J Dairy Sci. 2010;93(6):2639-50. DOI: 10.3168/jds.2009-3007
[7] Eckert E, Brown HE, Leslie KE, DeVries TJ, Steele MA. Weaning age affects growth, feed intake, gastrointestinal development, and behavior in Holstein calves fed an elevated plane of nutrition during the preweaning stage. J Dairy Sci. 2015;98(9):6315-26. DOI: 10.3168/jds.2014-9062
[8] Raeth-Knight M, Chester-Jones H, Hayes S, Linn J, Larson R, Ziegler D, et al. Impact of conventional or intensive milk replacer programs on Holstein heifer performance through six months of age and during first lactation. J Dairy Sci. 2009;92(2):799-809. DOI: 10.3168/jds.2008-1470
[9] D'Alessandro A, Zolla L, Scaloni A. The bovine milk proteome: cherishing, nourishing and fostering molecular complexity. An interactomics and functional overview. Mol Biosyst. 2011;7(3):579-97. DOI: 10.1039/c0mb00027b
[10] Bhattarai B, Fosgate GT, Osterstock JB, Park SC, Roussel AJ. Perceptions of veterinarians and producers concerning Johne's disease prevalence and control in US beef cow-calf operations. BMC Vet Res. 2014;10:27. DOI: 10.1186/1746-6148-10-27
[11] Hammon HM, Frieten D, Gerbert C, Koch C, Dusel G, Weikard R, et al. Different milk diets have substantial effects on the jejunal mucosal immune system of pre-weaning calves, as demonstrated by whole transcriptome sequencing. Sci Rep. 2018;8:1693. DOI: 10.1038/s41598-018-19954-2
[12] Schäff CT, Gruse J, Maciej J, Mielenz M, Wirthgen E, Hoeflich A, et al. Effects of Feeding Milk Replacer Ad Libitum or in Restricted Amounts for the First Five Weeks of Life on the Growth, Metabolic Adaptation, and Immune Status of Newborn Calves. PLOS ONE. 2016;11:e0168974. DOI: 10.1371/journal.pone.0168974
[13] Bach A. Ruminant Nutrition Symposium: Optimizing Performance of the Offspring: nourishing and managing the dam and postnatal calf for optimal lactation, reproduction, and immunity. J Anim Sci. 2012;90(6):1835-45. DOI: 10.2527/jas.2011-4516
[14] Khan MA, Weary DM, von Keyserlingk MAG. Invited review: Effects of milk ration on solid feed intake, weaning, and performance in dairy heifers. J Dairy Sci. 2011;94(3):1071-81. DOI: 10.3168/jds.2010-3733
[15] De Paula Vieira A, Guesdon V, de Passillé AM, von Keyserlingk MAG, Weary DM. Behavioural indicators of hunger in dairy calves. Appl Anim Behav Sci. 2008;109(2-4):180-9. DOI: 10.1016/j.applanim.2007.03.006
[16] Khan MA, Bach A, Weary DM, von Keyserlingk MAG. Invited review: Transitioning from milk to solid feed in dairy heifers. J Dairy Sci. 2016;99(2):885-902. DOI: 10.3168/jds.2015-9975
[17] Geiger AJ, Parsons CLM, James RE, Akers RM. Growth, intake, and health of Holstein heifer calves fed an enhanced preweaning diet with or without postweaning exogenous estrogen. J Dairy Sci. 2016;99(5):3995-4004. DOI: 10.3168/jds.2015-10405
[18] de Passillé AM, Rabeyrin M, Rushen J. Associations between milk intake and activity in the first days of a calf's life and later growth and health. App Anim Beh Sci. 2016;175:2-7. DOI: 10.1016/j.applanim.2014.10.002
[19] Ollivett TL, Nydam DV, Linden TC, Bowman DD, Van Amburgh ME. Effect of nutritional plane on health and performance in dairy calves after experimental infection with Cryptosporidium parvum. J Am Vet Med Assoc. 2012;241(11):1514-20. DOI: 10.2460/javma.241.11.1514
[20] Medrano-Galarza C, LeBlanc SJ, DeVries TJ, Jones-Bitton A, Rushen J, Marie de Passillé A, et al. A survey of dairy calf management practices among farms using manual and automated milk feeding systems in Canada. J Dairy Sci. 2017;100(8):6872-84. DOI: 10.3168/jds.2016-12273
[21] Jensen MB, Duve LR, Weary DM. Pair housing and enhanced milk allowance increase play behavior and improve performance in dairy calves. J Dairy Sci. 2015;98(4):2568-75. DOI: 10.3168/jds.2014-8272
[22] Rosenberger K, Costa JHC, Neave HW, von Keyserlingk MAG, Weary DM. The effect of milk allowance on behavior and weight gains in dairy calves. J Dairy Sci. 2017;100(1):504-12. DOI: 10.3168/jds.2016-11195
[23] Svensson C, Liberg P. The effect of group size on health and growth rate of Swedish dairy calves housed in pens with automatic milk-feeders. Prev Vet Med. 2006;73(1):43-53. DOI: 10.1016/j.prevetmed.2005.08.021
[24] Scholz AM, Bunger L, Kongsro J, Baulain U, Mitchell AD. Non-invasive methods for the determination of body and carcass composition in livestock: dual-energy X-ray absorptiometry, computed tomography, magnetic resonance imaging and ultrasound: invited review. Animal. 2015;9(7):1250-64. DOI: 10.1017/s1751731115000336
[25] Jesse GW, Thompson GB, Clark JL, Hedrick HB, Weimer KG. Effects of Ration Energy and Slaughter Weight on Composition of Empty Body and Carcass Gain of Beef Cattle. Journal of Animal Science. 1976;43(2):418-25. DOI: 10.2527/jas1976.432418x
[26] Chapman CE, Wilkinson PS, Murphy MR, Erickson PS. Technical note: Evaluating nuclear magnetic resonance spectroscopy for determining body composition in Holstein dairy calves using deuterium oxide dilution methods. J Dairy Sci. 2017;100(4):2807-11. DOI: 10.3168/jds.2016-11888
[27] Braun U, Krüger S, Hässig M. Ultrasonographic examination of the reticulum, rumen, omasum and abomasum during the first 100 days of life in calves. Res Vet Sci. 2013;95(2):326-33. DOI: 10.1016/j.rvsc.2013.03.019
[28] Braun U, Jacquat D, Hässig M. Ultrasonography of the rumen in 30 Saanen goats. Schweiz Arch Tierheilkd. 2011;153(9):393-9. DOI: 10.1024/0036-7281/a000231
[29] Esselburn KM, Hill TM, Bateman HG, 2nd, Fluharty FL, Moeller SJ, O'Diam KM, et al. Examination of weekly mammary parenchymal area by ultrasound, mammary mass, and composition in Holstein heifers reared on 1 of 3 diets from birth to 2 months of age. J Dairy Sci. 2015;98(8):5280-93. DOI: 10.3168/jds.2014-9061
[30] Nishimura M, Yoshida T, El-Khodery S, Miyoshi M, Furuoka H, Yasuda J, et al. Ultrasound imaging of mammary glands in dairy heifers at different stages of growth. J Vet Med Sci. 2011;73(1):19-24. DOI: 10.1292/jvms.09-0503
[31] Pietrobelli A, Formica C, Wang Z, Heymsfield SB. Dual-energy X-ray absorptiometry body composition model: review of physical concepts. Am J Physiol. 1996;271(6):E941-51. DOI: 10.1152/ajpendo.1996.271.6.E941
[32] Heymsfield SB, Hu HH, Shen W, Carmichael O. Emerging Technologies and their Applications in Lipid Compartment Measurement. Trends Endocrinol Metab. 2015;26(12):688-98. DOI: 10.1016/j.tem.2015.10.003
[33] Mandarim-de-Lacerda CA. Stereological tools in biomedical research. An Acad Bras Cienc. 2003;75(4):469-86. DOI: 10.1590/s0001-37652003000400006
[34] Kraybill HF, Bitter HL, Hankins OG. Body Composition of Cattle. II. Determination of Fat and Water Content From Measurement of Body Specific Gravity. Journal of Applied Physiology. 1952;4(7):575-83. DOI: 10.1152/jappl.1952.4.7.575
[35] Jones SDM, Price MA, Berg RT. The density of Bovine limb bones. Can J Anim Sci. 1978;58(1):105-6. DOI: 10.4141/cjas78-014
[36] Buehler T, Ramseier N, Machann J, Schwenzer NF, Boesch C. Magnetic resonance imaging based determination of body compartments with the versatile, interactive sparse sampling (VISS) method. Journal of Magnetic Resonance Imaging. 2012;36(4):951-60. DOI: 10.1002/jmri.23707
[37] Hu HH, Chen J, Shen W. Segmentation and quantification of adipose tissue by magnetic resonance imaging. Magma. 2016;29:259-76. DOI: 10.1007/s10334-015-0498-z